VIDEOS
We re-edited our published research into short videos to make them easier to understand!
As a new non-clinical trial model using human cells, organoids and organ-on-chips are being developed worldwide. However, a system that incorporates both technologies at the same time faces a significant challenge to practical application due to the complexity of the experiments.
The system we have developed modularizes three-dimensional tissues, including organoids, into cube-shaped culture vessels. This enables the technology of organoid culture to be incorporated into microfluidic chips, creating a platform that can easily implement organ-on-chip technology.
By creating various types of cell or tissues in advance within the cube, we can construct an in vitro experimental model system that can easily combine multiple organoids and express intercellular and inter-organ interactions.
Here, we present a workflow for culturing organoids with morphogen gradient using a CUBE culture device, followed by sectioning samples with the CUBE to retain information on gradient direction. We show that hiPSC spheroids cultured with two separated differentiation media on opposing ends of the CUBE resulted in localized expressions of the respective differentiation markers, in contrast to homogeneous distribution of markers in controls. We also describe the processes for cryo and paraffin sectioning of spheroids in CUBE to retain gradient orientation information. This workflow from gradient culture to sectioning with CUBE can provide researchers with a convenient tool to generate increasingly complex organoids and study their developmental processes in vitro.
Localization of multiple hydrogels is expected to develop the structure of 3D tissue models in a location-specific manner. To achieve the localization, a unit-based scaffold (MultiCUBE) is developed with a unique frame design to trap hydrogel solutions inside their designated units. An optimal range of unit dimensions and surface wettabilities enables a solution trapping up to several cubic millimeters without any need for chemical additives. This capability allows spatial organization of biomolecular compositions and physical conditions of hydrogels, as well as the relative position of biological samples (cells, spheroids, and reconstituted tissues) within the scaffold.
To investigate how the extracellular matrix (ECM) that surrounds cells affect cellular behaviour, as well as how cells themselves influence their surrounding ECM, we tracked the movement of cells under two conditions: without a Matrigel cover and with a Matrigel cover. What we observed was that, in the condition with a Matrigel cover, cells created a path in the gel as they travelled, and other cells behind preferentially followed the same trail left behind by the cell in front. When we stained and imaged the path in the gel, we found that the path was actually a 3D tunnel. From these observations, we can see that cell movement is initially restricted due to resistance force from the surrounding ECM, but as the cells begin to degrade and weaken the resistance of their surroundings, other cells easily follow along the path with weakened resistance.
We constructed a mathematical model with the assumption that the weakening of ECM resistance force is due to the physical interaction between cells and ECM, and confirmed that the simulation results matched with the experimental results. Therefore, we hypothesized that we could control the direction of cell movement by controlling the resistance force of the cell’s surroundings – that is, by mixing regions of high resistance with that of low resistance. To prove this hypothesis, we placed magnetic beads in a small area close to the cell population and used a permanent magnet to weaken the surrounding ECM by dragging the magnet back and forth, causing the magnetic beads to create “paths” in the gel. As we had predicted, the cells actively migrated in the direction where resistance had been weakened, in both experimental and simulation results.
Cells appear to move randomly when simply seeded on a dish, but when the cell population is seeded in a fixed shape, we begin to see a very unique and highly reproducible pattern in their movement. For example, when bronchial epithelial cells were seeded in a triangular shape made by micromachining process, the cells always moved toward the apexes of the triangle first, before bouncing back towards the middle to re-form the triangle, and finally exiting the triangle from the apexes. The fact that the cells always move in the same manner implies that there are certain rules that determine the direction in which cells move. If we can hypothesize these rules and incorporate them into mathematical models, we will be able to analyse spatiotemporal changes that cannot be observed in experiments alone. Therefore, this approach can open up the possibilities to deepen our understanding of cell behaviour by logical methods.
The gel walls of the Cube culture device provide sufficient rigidity that the 3D-cultured sample can easily be picked up and rotated by tweezers, but at the same time still allows nutrients to pass through the network structure of the gel to the tissue sample inside. By taking advantage of this easy handling ability, various engineering technologies can be packed into the Cube. For example, until now it was difficult to image whole samples with high sensitivity/resolution using low-magnification lens, but by rotating the Cube and scanning the sample from all 6 directions, we are able to dramatically improve the quality of imaging even with the same microscope and same sample.
When bronchial epithelial cells are dispersed within Matrigel, they only form a cyst structure and do not branch; but when seeded as a cluster of cells in the gel, the cells initially aggregate tightly together before forming branches. Furthermore, when vascular endothelial cells (HUVEC) are dispersed in the Matrigel surrounding the bronchial epithelial cell cluster, longer and more highly branched structures formed over a period of time. When observed over the course of the branching process, we found that the cells go through the processes of 1) aggregation, 2) development of leader cells, 3) disappearance of leader cells and contraction of the branches, 4) branching and elongation by spiralling movement of cells, 5) maintenance of branches.
Journal Covers
Photos


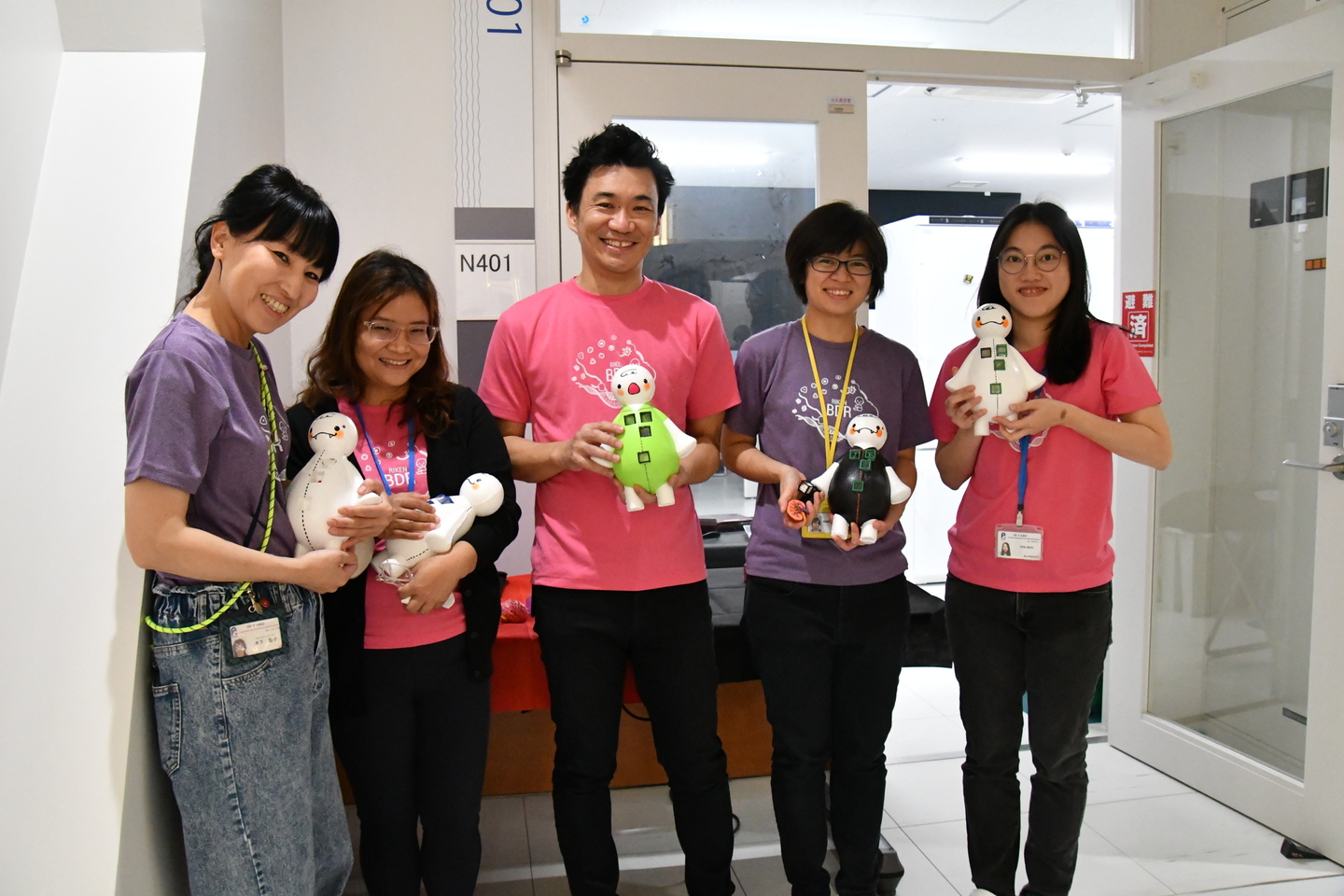
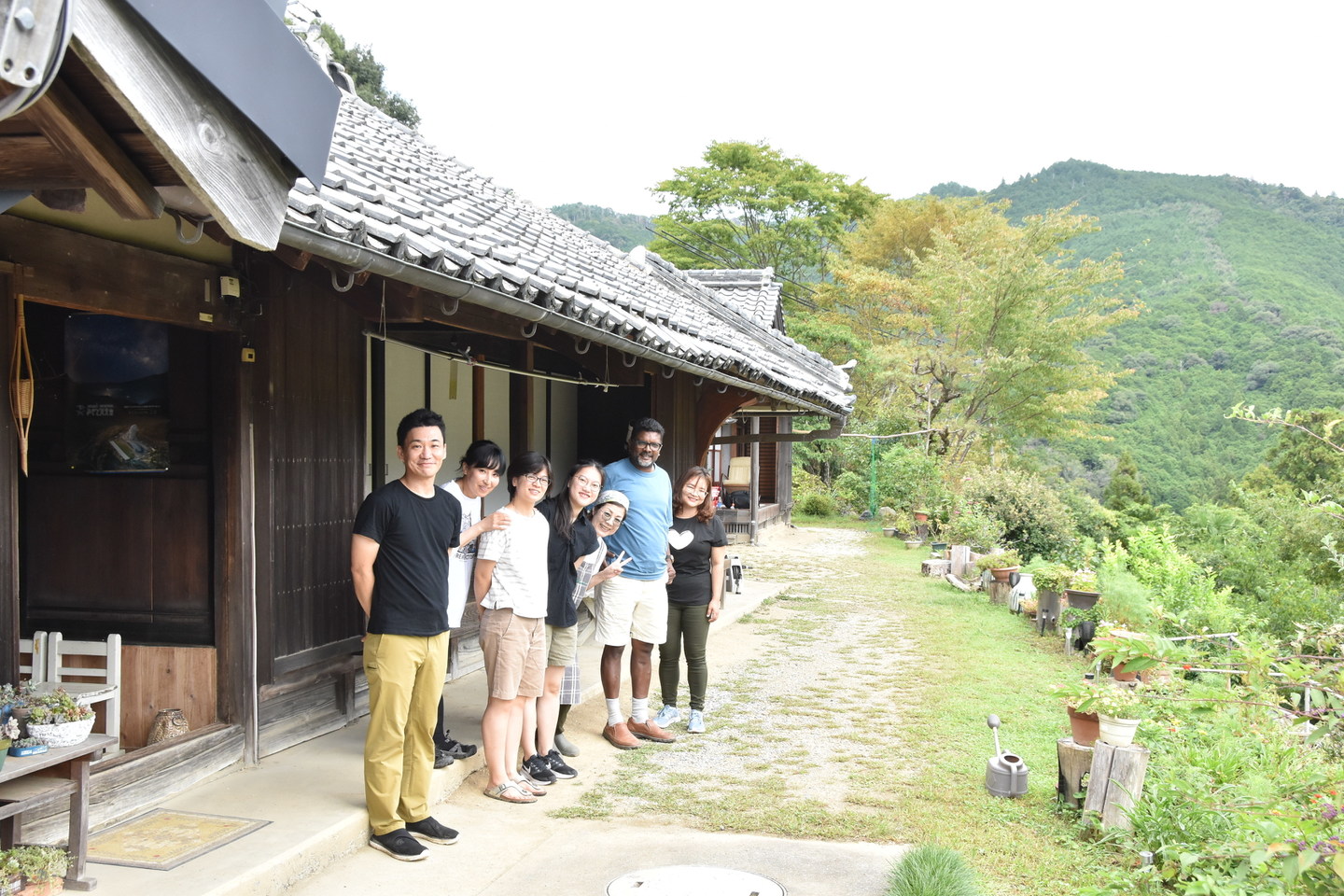
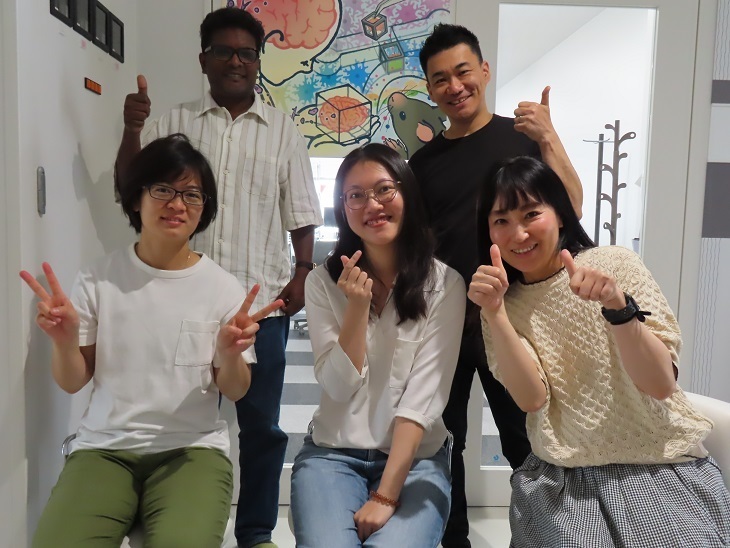
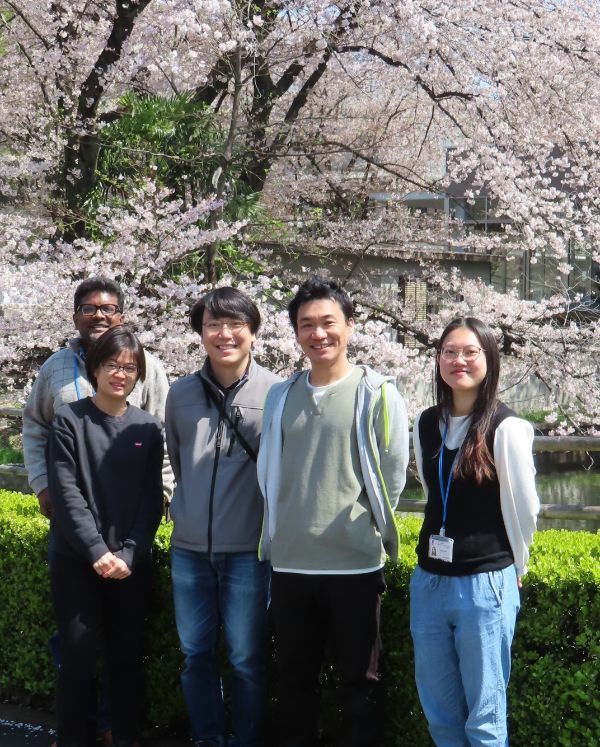
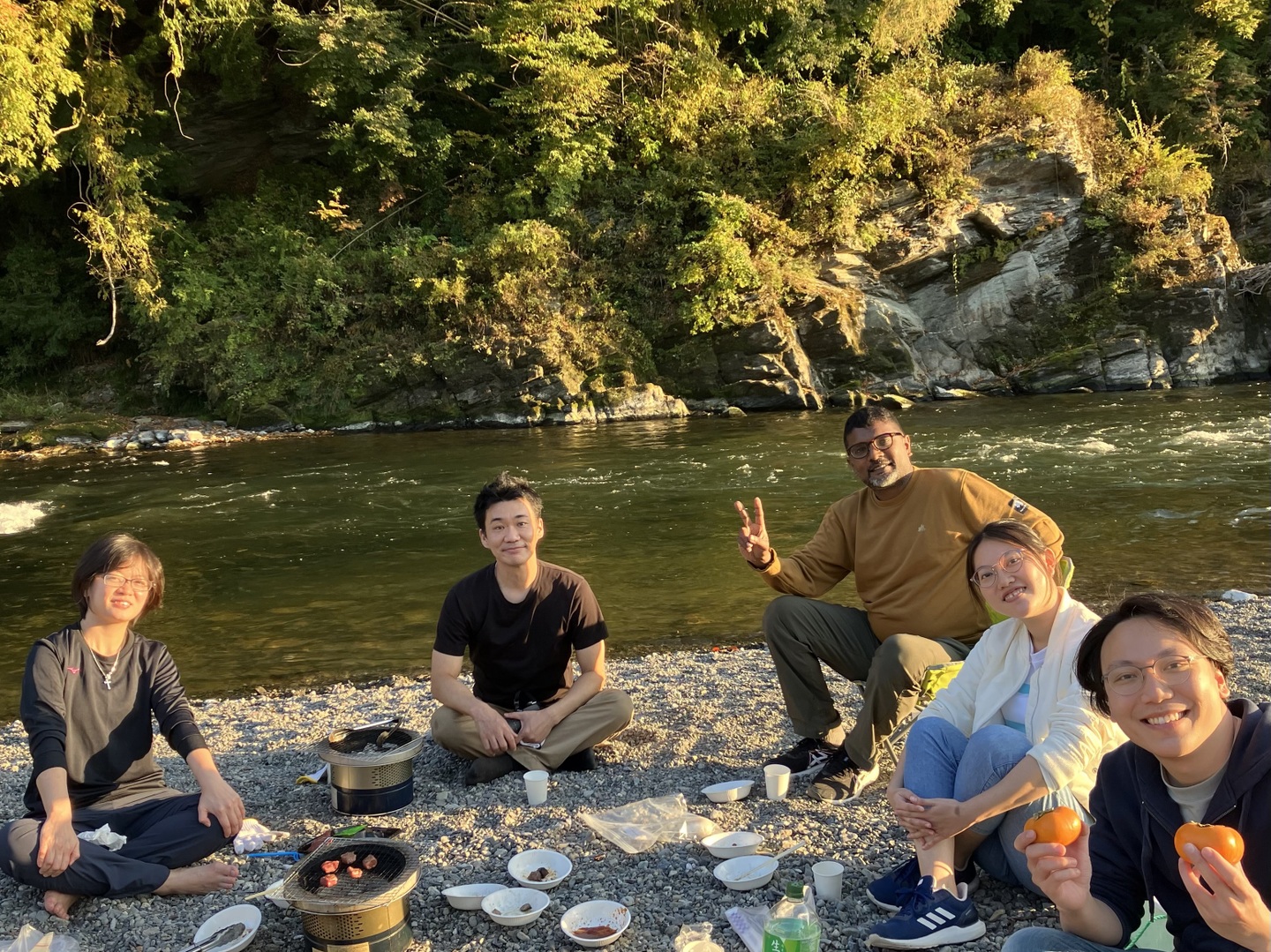
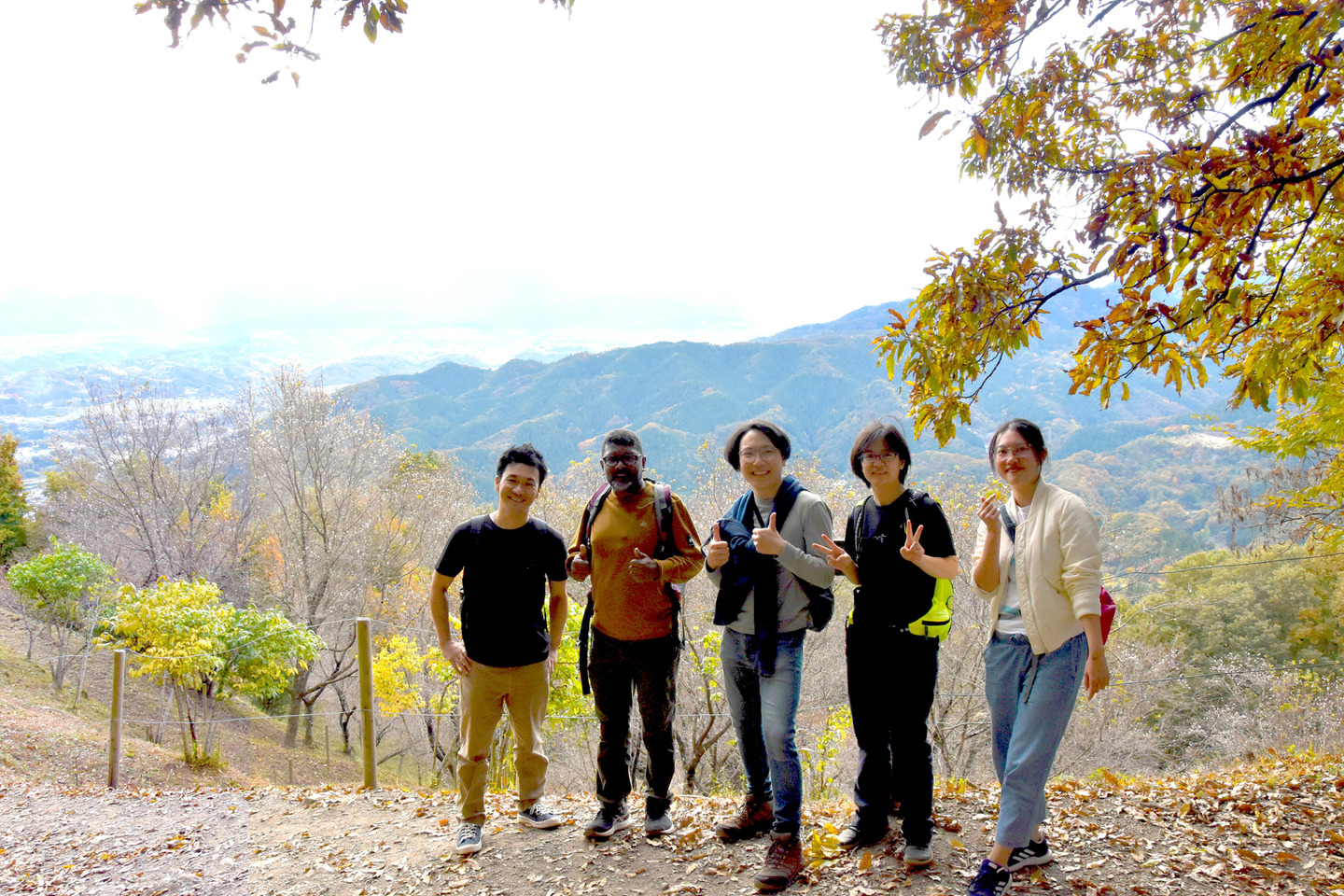
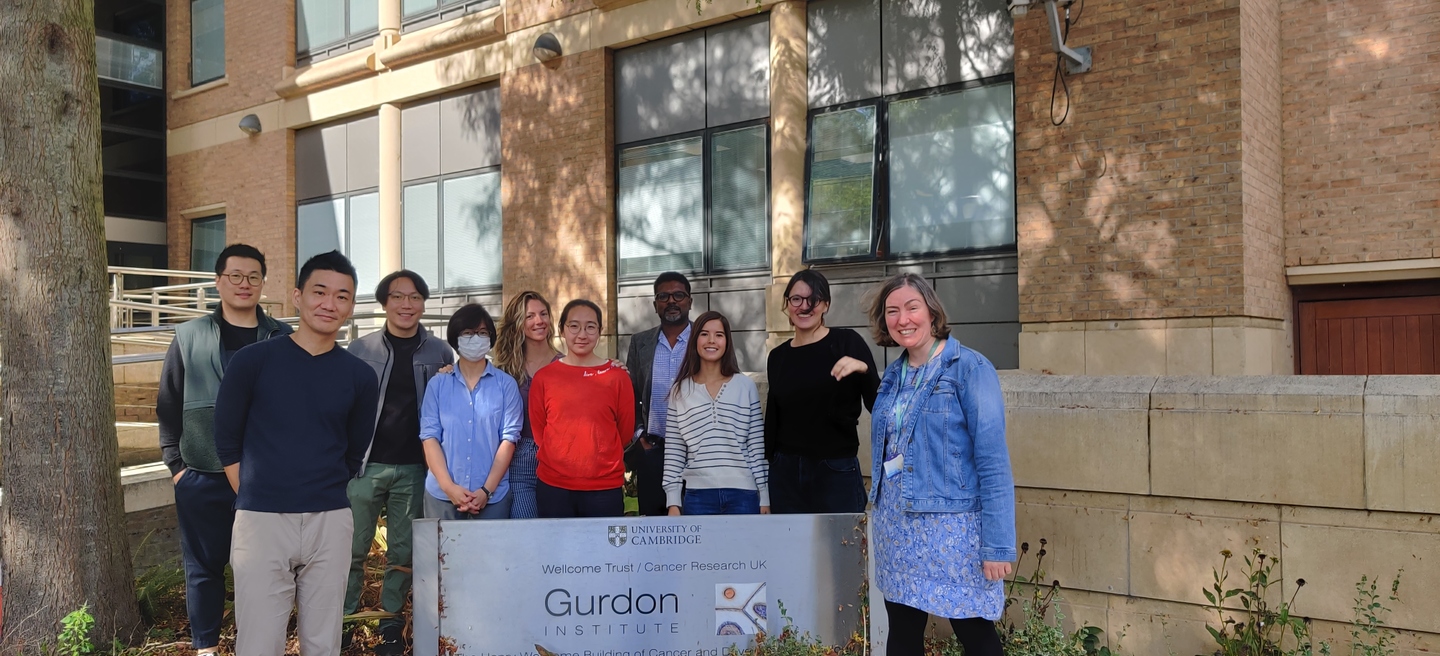
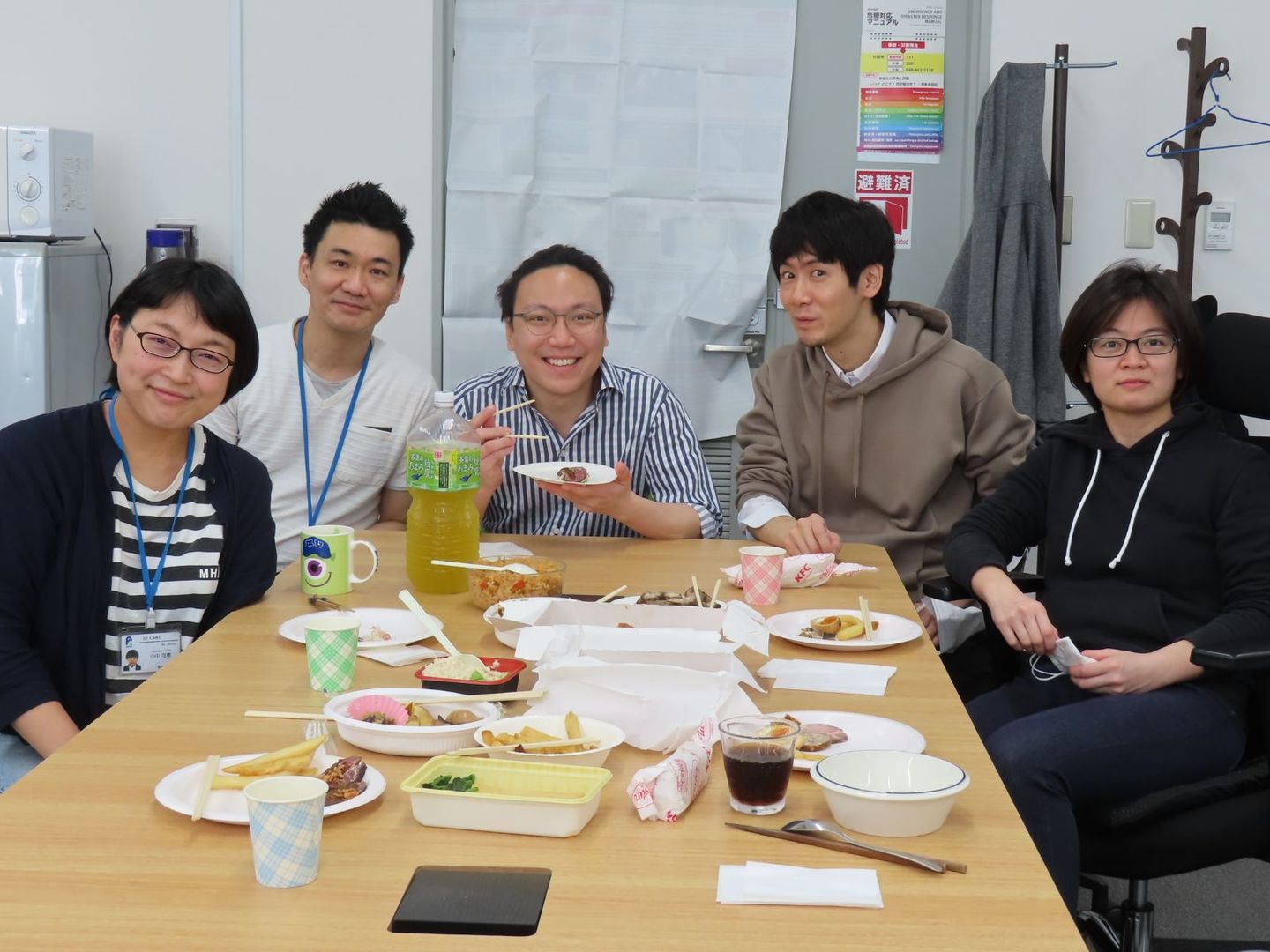
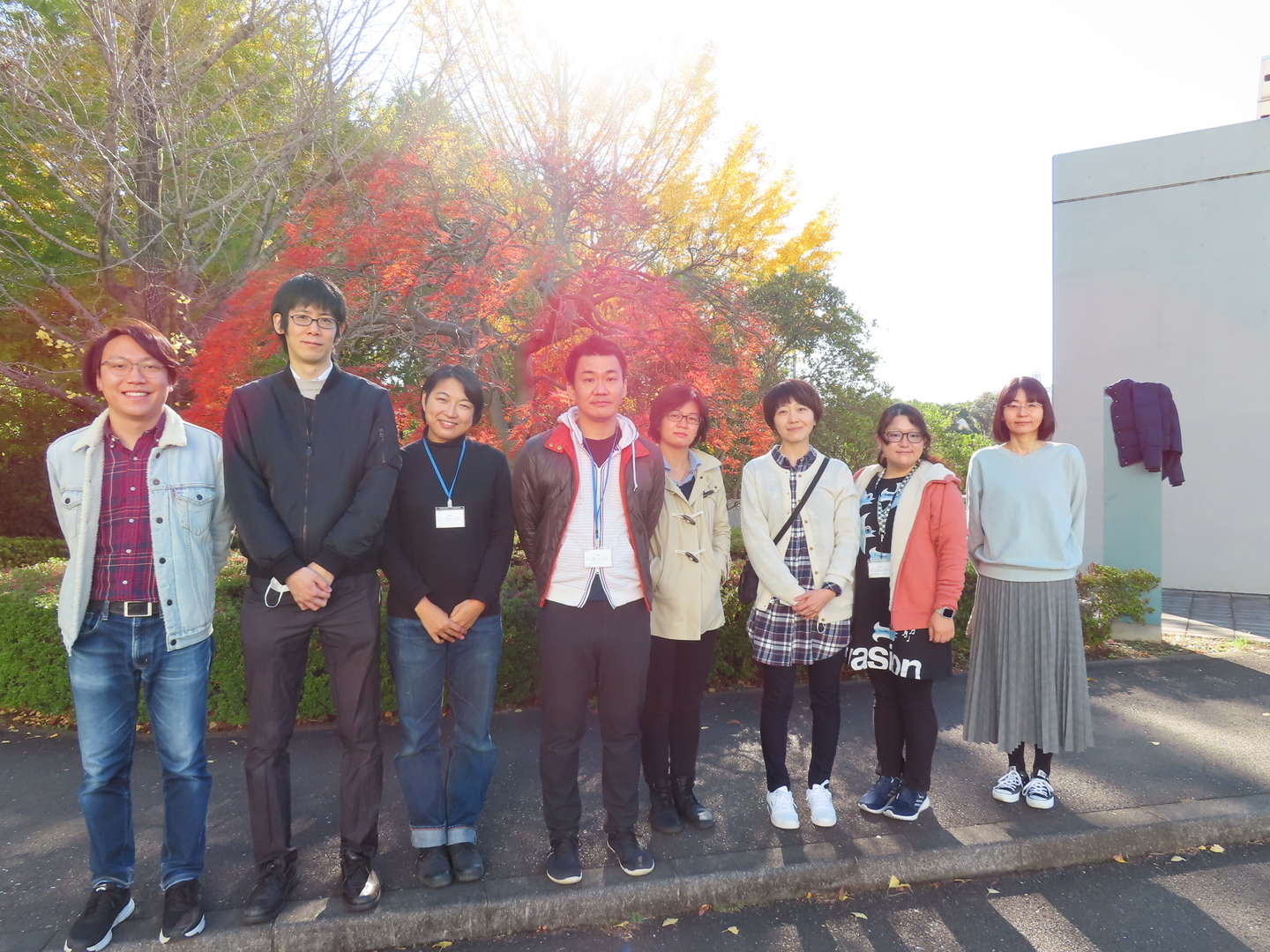