CONCEPT
Synthesis of different approaches makes something unique.

Challenge of Reconstructing In Vivo Environments In Vitro
Organs possess distinct macro anisotropic structures, and within each organ, different regions are induced to differentiate and function uniquely. However, many organoids, cultured outside of body from stem cells, are formed under spatially uniform conditions, relying on the autonomous organization of cells that have lost positional information. As a result, these organoids are typically spherical in shape, with differentiated cells scattered within. Such an approach makes it exceedingly challenging to replicate the original structure of an organ.
To reconstruct the macro anisotropic structures of organs in vitro, we believe it is essential to create a "field" where cells can recognize positional information, similar to the in vivo environment, and guide the autonomous organization of cell populations in the desired direction. However, given the impossibility of fully reproducing the complexity of the in vivo environment, where hundreds of factors intricately interact, we must establish theories and techniques specific to in vitro environments for designing and controlling such fields to reconstruct complex organ structures.
In response to this challenge, we aim to develop methodologies for designing and controlling culture environments that enable the autonomous organization of cell populations into targeted macrostructures. Our goal is to develop technologies to spatially and temporally control the necessary environmental factors, thereby reconstructing the complex morphology of organs in vitro.
Organoid architechture control through spatial regulation of cells, ECM, and growth factors

Reconstruction of Neural Tube Formation Processes via a Body Axis Formation Platform
During embryonic development, cells receive positional information for the dorsal-ventral, anterior-posterior, and left-right body axes even in the early stages. This spatial information determines cell differentiation and function, enabling the asymmetric formation of organs. In neural tube formation, in particular, cells undergo mechanochemical feedback to establish precise differentiation patterns along the dorsal-ventral and anterior-posterior axes, which play a crucial role in subsequent developmental processes.
We aim to achieve this by using a modularized device that controls the surrounding environment to deliver the appropriate spatiotemporal information required for body axis formation. This approach focuses on reconstructing the neural tube closure process and the subsequent induction and formation of differentiation patterns in vitro.


Application of Reconstructed Barrier-Function Tissues to Pharmacokinetic Models
The human body possesses numerous barrier-function tissues that prevent external substances from easily entering the body. One such barrier is the blood-brain barrier (BBB), where brain-surrounding blood vessels are more robustly structured than other vessels to protect the brain. However, this protective function of the BBB poses a challenge: delivering drugs effectively to the brain.
To address this, we successfully recreated key BBB functions, such as tight junctions and transporters, by co-culturing brain microvascular endothelial cells differentiated from human iPSCs with primary astrocytes and pericytes that support endothelial cells. This was achieved using our CUBE system.
Similarly, we developed a skin model with barrier functionality by co-culturing human keratinocytes and fibroblasts derived from human skin within the CUBE system, allowing for keratinization.
These tissue modules with barrier functionality can be applied to pharmacokinetic testing to evaluate the extent to which candidate drug compounds can penetrate these barriers. Furthermore, the modular nature of these tissues facilitates their integration into microfluidic chips. This modular approach enables the combination of barrier-functional tissues with other organoid models, allowing for the exploration of inter-tissue interactions. We are currently advancing the construction of various interaction models to expand the applicability of this platform.
Analysing Self-organized Formation Mechanism from Cells to Tissue Pattern
When cells are cultured under homogeneous conditions in a dish, it is difficult to recognize the underlying rules governing cell behaviour because even small variations in the conditions of a cell’s vicinity (for example, distance between cells) can lead to differences in cell state and behaviour. This variation is exacerbated/greatly increased during the growth phase of a population of cells, which leads to major deterioration of the reproducibility of results. However, we believe that this variation can be suppressed by controlling the culture environment, and by systematically introducing perturbations to the culture environment, we can unravel the rules governing cell behaviour. For example, if a population of bronchial epithelial cells are seeded in a triangular shape in a dish under specific conditions, the cells will always initially move toward the apex of the triangle; on the other hand, if seeded in a circular shape, the cell population will rotate in the circle as a whole. This means that the direction of cell movement changes depending on the boundary conditions of the cell population. Then, what are the changes due to modifications in boundary conditions that affect cell behaviour? By pursuing these questions, we can understand the rules governing cellular behaviour in more detail.
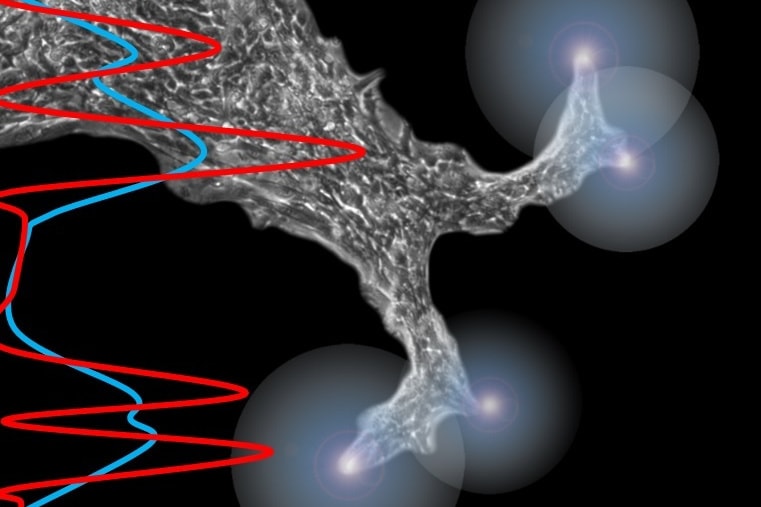
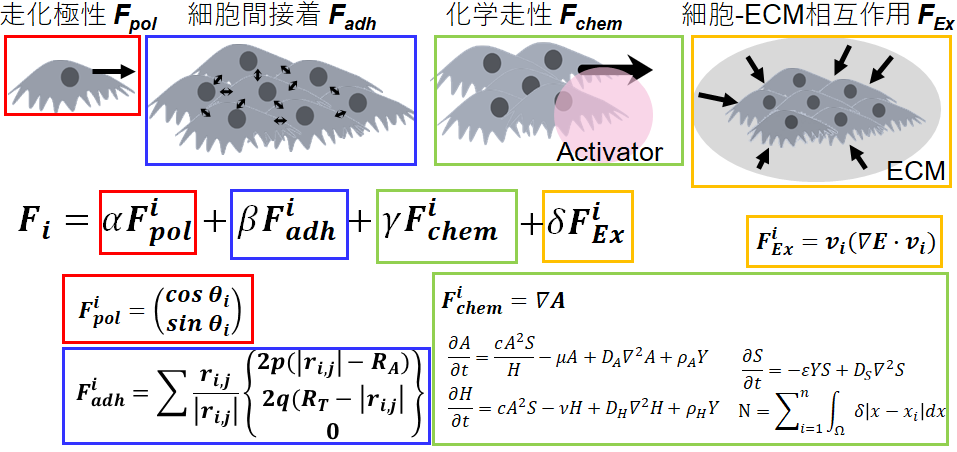